Carbon’s Lifeless Cycle: A Geologic View of Climate Stability And Change
- Boopalakrishnan Arul
- Jun 7, 2024
- 10 min read
BY BOOPALAKRISHNAN ARUL
Karstic limestone in the Spanish site of El Torcal de Antequera. The rock in this high outcropping was formed on a Jurassic-era seabed, 150 million years ago, Wikimedia Commons.
In 2013, the combustion of energy-rich hydrocarbon fuels, in sectors like transportation, electricity, and heating, accounted for 72% of global greenhouse emissions. Most of this came in the form of carbon dioxide. The respiration of simple sugars (another energy-rich hydrocarbon fuel) by archaea living on the vast acreage of anoxic agricultural environments (such as traditional rice paddies, or the intestines of livestock) contributed another 11% of total emissions, mostly in the form of methane.
These two gases are the heat-trapping mechanisms of climate change. Their accumulation in the atmosphere is a direct consequence of how human societies deal with Earth’s life and its remains. Photosynthesis turns airborne carbon dioxide into fuel: long, heavy, kinked organic molecules full of bonds which can be broken for energy. Deposit after deposit of this fuel has been consumed over the last century, and combustion turns it all into airborne, heat-trapping carbon. Meanwhile, marine and terrestrial ecosystems that store carbon have been degraded by extractive land and water use.
The proportion of heat-trapping molecules in the atmosphere continues to rise. In 2023, estimates of the global atmospheric carbon dioxide converged on 419.3 parts per million (ppm) by mass, around 50% greater than in 1750. The maximum ratio of carbon dioxide in the air has never exceeded 300 ppm for the last million years, except in the last three centuries. The result has been a heating of the entire earth by one degree Celsius over the last fifty years.
An integrated solution might involve restoring ecosystems that withdraw carbon from the atmosphere, changing how energy is accessed for human needs, and adjusting agricultural practices to avoid replacing carbon-sequestering ecosystems with methane-producing systems. It’s a popular package of ideas. In 2020, the United Nations Development Programme asked 1.2 million people from 50 countries how they would rank three suggested policies in each of six topic areas (“To address the climate crisis, what should your country do about…”). The three most popular policies were conserving forests and land (prioritized by 54% of respondents), producing more renewable energy (53%), and using climate friendly farming techniques (52%).
The survey's predetermined options, however, omit a key detail: carbon is more than just fuel. Big, combustible organics are not the only carbon reservoir exploited for human demands. Industrial processes, such as concrete and steel production, consume mineral reservoirs of carbonates. In 2013, the byproducts of industrial processes accounted for 6% of total greenhouse emissions, totaling over half the impact of all agriculture.
As emissions from multiple sources mingle in the atmosphere, they participate in an ongoing planetary cycle of inorganic carbon, weaving through the states of nonliving matter. Over four billion years of Earth’s history, the inorganic carbon cycle has been the most effective force for withdrawing carbon from the atmosphere. It has helped ensure Earth’s existence as a planet of liquid water and teeming life, and it has conditioned the history of life on Earth from its earliest days. If the inorganic carbon cycle is not integrated into efforts to resolve climate change, it will continue pushing the planet toward its own version of stability, which may not align with our preferences. If that happens, even a revolution in energy sources and agricultural practices will not forestall drastic changes to Earth’s ecosystems.
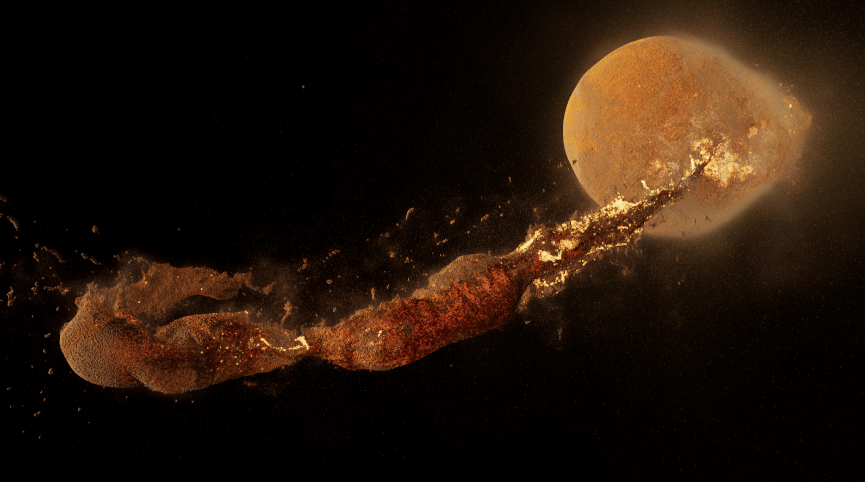
Simulation of the giant-impact hypothesis of the Moon’s formation, NASA Ames Research Center.
The last million years, the present industrial moment included, have been very stable compared to how things were four billion years ago, when the Earth had a surface temperature over two thousand degrees Celsius. After colliding into a body one tenth of its present size, the planet liquefied, absorbing the kinetic energy of the impact as a tremendous quantity of heat. Carbon dioxide and water, which lose their solubility in high-temperature magma, bubbled out and created a new superheated atmosphere with a pressure hundreds of times greater than the present 1.01 bars. Carbon dioxide and water vapor are very effective greenhouse gases. The earth was trapped with its excess heat, which slowly escaped into space over millions of years. How did a planet like that, with its Venus-like conditions, give birth to life?
The creation of the Earth, asteroid impacts that shuffled and reshuffled its makeup, and the emergence of life on its surface, all fall into the Hadean Eon, the most ancient period of Earth’s life. Geologists cataloging the few surviving specimens of this era, the three- or four-billion year old rocks from the hard, ancient cratons at the centers of the continents, set the boundaries of the Hadean at 4.5 and 3.8 billion years ago. With very little material left to examine, attempts to understand global conditions on the Hadean Earth use physical and chemical models, simulating the practical consequences of theoretically grounded assumptions.
The major player in these simulated earths is a carbon cycle— not the organic carbon cycle which places ourselves, other life, and fossil fuels at the center of events, but the inorganic carbon cycle of gas mixtures, fluid solutions, and minerals. To understand how it could drive an entire planet toward temperate and habitable conditions, it may help to observe how it transforms a single rock, grain by grain.
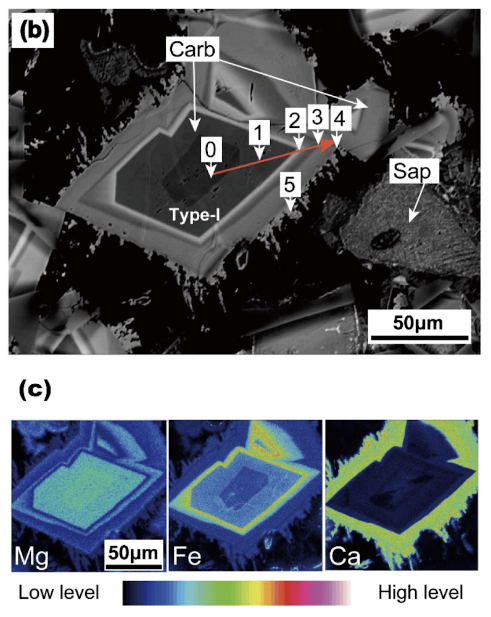
Secondary magnesium, iron, and calcium carbonates formed by the reaction of komatiite with a CO2-rich fluid at high pressure and temperature, Ueda and Shibuya 2021.
Current assumptions about the early Hadean have a surprising implication: the planet’s surface may have been completely immersed in an exotic state of matter. Beyond a critical point (374 degrees C and 221 bars of pressure for water, 31.2 degrees Celsius and 74 bars for CO2), a fluid will go supercritical, expanding to fill its container (like a gas) but also dissolving solutes within itself (like a liquid). In the early Hadean, the sheer quantity of greenhouse gases released from the steaming Earth may have created a supercritical atmosphere: a fluid mass of hybrid properties, whirling over a black, igneous crust of basalt and other rocks rich in metal oxides.
An experiment from 2021 simulated this early Earth by putting igneous rocks in contact with a supercritical “atmosphere” of hydrochloric acid, water, and CO2. The rocks dissolved and re-crystallized into two varieties of secondary minerals. Negatively charged carbonate ions bound to positively charged metal (magnesium, iron, calcium) ions and crystallized out of the solution, with different pairings dominating at particular temperatures. Simultaneously, silicates like quartz (pure silica, SiO2) and clays (interleaved hydrated sheets of silica and metal oxides) formed around the carbonates.
This was a highly effective method of carbon capture: atmospheric greenhouse gas was locked inside enormous deposits of minerals, which floated on the growing continents or sank to the seafloor, disappearing under the sliding tectonic plates. On a scale of millions of years, the trade of positively charged metal ions between carbonates, silicates, and oxides diversified the geology of the earth, enriching this ball of basalt with never-before-seen colors, textures, and hardnesses. As carbon dioxide exited the air, the remaining atmosphere trapped less heat and exerted less pressure, and the surface began to host liquid water.
This cooling force guided the planet through the devastating impacts of Hadean asteroid bombardments. Each impact superheated the Earth for thousands of years, boiling the oceans and vaporizing large chunks of the crust. This introduced volatile, energized particles of rock into the air or water, where they reacted with carbon dioxide and formed carbonate minerals. When the excess heat of the asteroid impact dissipated, the Earth wound up even cooler than before. A comparison of models showed that while leaving out the effect of asteroid impacts would support the preconception of a hot and acidic Hadean, factoring the effect in led to revised estimates of cold, even sub-freezing global average temperatures, mainly caused by a large decrease in estimated atmospheric CO2.
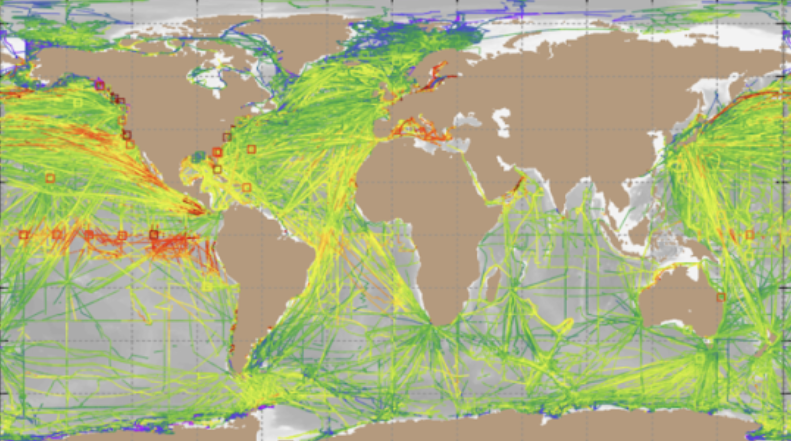
Ocean CO2 as of 2023, measured by ships, buoys, and autonomous platforms. Surface Ocean CO2 Atlas.
By the same principles that shaped the Hadean world, shouldn’t it be possible for the excess CO2 of modern times to be reconstituted into new mineral reservoirs? This is already happening: the ocean absorbs 31% of CO2 released into the air. First, atmospheric CO2 diffuses into water and reacts with it to form carbonic acid (H2CO3), which in turn reacts with metal rich rocks in the supercritical fluid-filled channels of “black smoker” hydrothermal vents. These high-temperature, high-pressure crucibles form new minerals, with carbon atoms locked tightly into their crystal structures. Organisms with shells or exteriors of calcium carbonate, like diatoms, sea urchins, corals, or mollusks, also contribute greatly to the crystallization of carbon.
In the meantime, however, all the extra carbonic acid has decreased the entire ocean’s average pH by 0.1 (converting from the log scale, this means a 26% increase in the concentration of H+) in the last 200 years. Existing systems of geochemical buffers will likely keep the ocean alkaline overall, but airborne CO2 molecules turning into dissolved ions en masse have already changed the chemical properties of the ocean.
Temperature and pH are similar, in that seemingly small changes in the global average may be more than life on earth is ready for. Acidification decreases the concentration of the materials that coral and shellfish use to build their shells and biostructures. It inhibits the normal methods by which phytoplankton take in iron, forcing them to limit iron consumption and explore alternate methods of taking in this vital nutrient. Half of the world’s primary production (the photosynthetic production of organic molecules from carbon dioxide) is accomplished by phytoplankton, highly efficient chemical factories vulnerable to shortages of critical inputs. Marine regions where iron is not available to phytoplankton end up classified as “high nutrient, low chlorophyll”, sparsely populated regions that cannot achieve their full productive potential. On land, acidic runoff can dissolve clays, disrupting their chemical structure of interleaved silicon and aluminum oxides. Runoff carries away aluminum atoms and concentrates them to harmful levels, fraying the dense interconnections between plants, microorganisms, and animals.
In the Hadean, extinction was part of the game. Bottlenecks of heat, cold, acidity, alkalinity, and other adversarial conditions pruned away the false starts of life. The survivors possessed a world-conquering balance of fidelity and flexibility in resource use and reproduction. As a distant consequence of all that, we now live here today. However, we cannot rely exclusively on Hadean-style carbon capture anymore. Losses of keystone species can send entire ecosystems into unsalvageable decline. Over ninety percent of the Great Barrier Reef’s corals have suffered a fatal bleaching in the last decade due to a number of factors, including rising ocean temperatures. Without the reefs to offer food and refuge, half of the world’s reef fish species may be at risk of extinction. Recovery may become impossible if acidification results in brittle corals unable to resist the pressures of erosion and grazing animals, or the shocks of powerful storms.
Does this mean that the inorganic carbon cycle is a threat to life on Earth? If its consequences are now threatening, it is only because of the conditions we have set up for it. Just as life looks for niches to expand into, chemical cycles look for imbalances, differences which can be brought to equilibrium. As the atmosphere is saturated with carbon, the ocean absorbs some of the excess. This is not a force we can ignore, much less one we can resist.
Understanding climate change solely in terms of life and its remains leads to a blinkered perspective on how carbon moves in and out of the atmosphere. In 2021, a United Nations survey of 700,000 respondents in the G20 countries found that the most popular policy, among respondents over and under 18, was the conservation of forests and land. This is still an important goal, but it’s important to remember that the majority of the carbon that once filled the Hadean atmosphere was sequestered by mineralization, not the creation of organic molecules. And alongside the fossil fuels exhumed from their crypts, vast mineral reservoirs of carbon are also rapidly being consumed.
The mass of human produced materials— concrete, steel, and plastics, and more— now exceeds the combined mass of all life on earth. A key ingredient of concrete production is quicklime (calcium oxide), which is produced by heating limestone (calcium carbonate) until CO2 is evicted from its mineral structure. In iron production, lime produced in exactly the same way is introduced to clean up impurities. Concrete and iron are the bulky mass of our society, present in our homes, transit, and technology. It’s enough of a challenge to separate oil and coal from our lives, and it is still harder to imagine a life without concrete and steel.
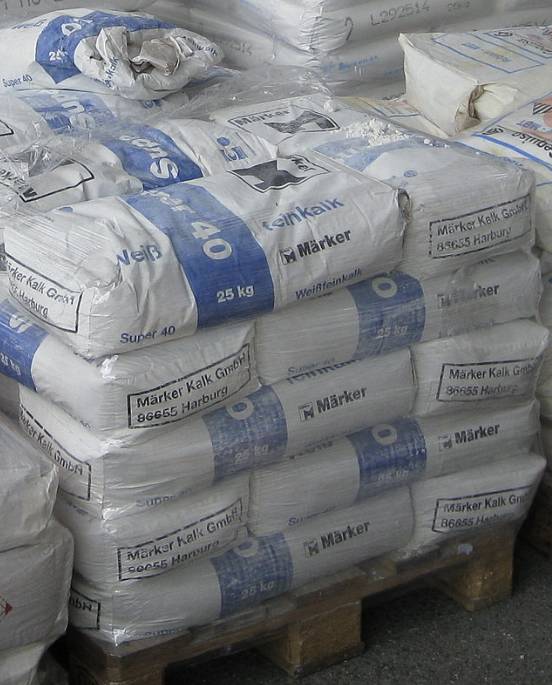
Bags of lime (calcium oxide), a key ingredient of concrete and steel. Limestone (CaCO3) is heated in kilns to produce CaO, releasing CO2. Wikimedia Commons.
Industrial and agricultural processes must be structured to capture CO2 byproducts or avoid releasing them in the first place. Direct reduced iron production is gaining ground as an alternative to methods that use natural gas or coal to make metallic iron. The CO2-heavy waste gases from cement production can be passed through solvents that selectively absorb and let go of CO2 in controlled conditions. Proposals for large-scale engineering of the environment are graduating from theory to practice: introducing a base like lime or calcium hydroxide is being tested as a way to counteract ocean and soil acidification. However, the main method of lime production is heating calcium carbonate, a process which releases CO2. The processes of production will determine whether geoengineering, even if it works as intended, is actually “carbon capture” or just carbon musical chairs.
Carbon dioxide and methane are some of the simplest forms which carbon can take. They are light enough to take to the air, and heavy enough to remain in the atmosphere. The Earth is covered in chemical loops that take in or release these molecules, and each system yields different perspectives on how climate change and stability come about. It is not a surprise that people have been receptive to the ecological angle. From this perspective, ecological understanding becomes a means of comprehending the world and thinking of practical ways to stabilize and preserve it.
But if life alone cannot maintain an optimal concentration of atmospheric carbon, non-life will pick up the slack. Since the Hadean Eon, chemical gradients have driven unbalanced concentrations of compounds toward a new equilibrium. The resulting conditions may be selective in the evolutionary sense, but they are an additional source of adversity for pivotal marine and terrestrial organisms. Instead of fighting a cycle older than life itself, approaches to climate change must, at minimum, consider its independent effects. By treating the interactions between man-made environments and the wider planet— the way our choices echo through the rock, sea, and air— as this century’s main engineering challenge, we may discover new advances and efficiencies. Beginning to think geologically also has its own reward: mute matter will seem to come alive, to have always been ebbing and flowing underfoot.
Коментари